ELY KOVETZ
SENIOR LECTURER
BEN-GURION UNIVERSITY
Opening Up New Windows to Study the Universe
The Standard Model of Cosmology aims to explains the cosmic evolution from a fraction of a second after the Big-Bang singularity to the current period of accelerated expansion with only a handful of parameters. Over the past decade, it has withstood a wide series of observational tests. Yet several gaping holes remain in the theory:
– How did inflation begin and come to an end?
– What makes up the dark matter in the Universe?
– What is the nature of dark energy?
– How did galaxies and clusters of galaxies form and evolve to make up the large scale structure we observe today?
Going forward, we must develop new ways to probe these fundamental questions by accessing the full volume of the observable Universe. This is the focus of my research.
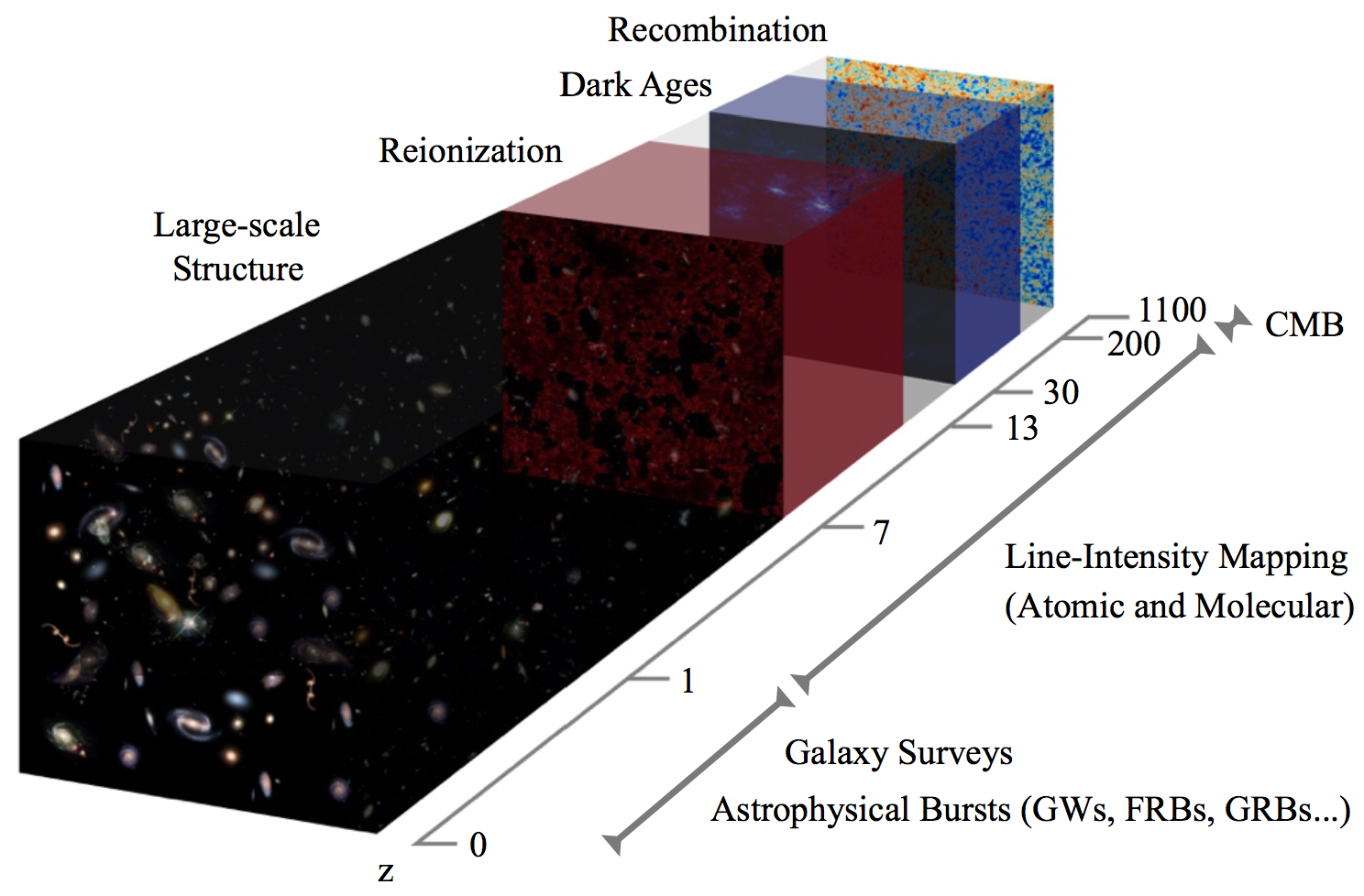
The Cosmic Microwave Background: Exploring the Early Universe
Why am I interested?
My interest in the CMB has been focused on cosmological Inflation, our best theoretical framework to explain why the Universe is flat, homogenous and isotropic, and most importantly how tiny quantum fluctuations are imprinted onto cosmic scales to provide primordial seeds for structure formation. The anisotropies in the CMB temperature can be used to test inflationary scenarios in various ways. Above all, the curl component (or B-mode) of the CMB polarization, a unique imprint of inflationary tensor fluctuations (or primordial gravitational waves), is a key prediction of many models of Inflation and is widely considered the holy grail of cosmology, as I reviewed in Kamionkowski and Kovetz (ARAA, 2016).
What is it about?
My focus of late has been on forecasting the ability of upcoming CMB experiments to probe a variety of features related to Inflation and Dark Matter.
With J. Munoz and colleagues, we laid out a novel second-order estimator of compensated isocurvature perturbations (Munoz et al. 2016) and investigated how well the running of the spectral index can be constrained (Munoz, Kovetz et al. 2017). Recently, with colleagues at JHU, IAS and Tel-Aviv, We performed an improved CMB analysis to search for dark matter–baryon scattering with a Rutherford cross section (a velocity dependence of v^-4) corresponding to a Coulomb-type interaction. In particular, we developed a new and robust prescription for incorporating the relative bulk velocity between the dark matter and baryon fluids into the standard linear Boltzmann calculation. Using an iterative procedure, we self-consistently include the effects of the bulk velocities in a cosmology in which dark matter interacts with baryons. Furthermore, we investigated how these constraints change when only a subcomponent of dark matter is interacting, with important implications for the dark-matter explanation of the recent anomalous 21cm absorption profile detected by the EDGES experiment at z~17.
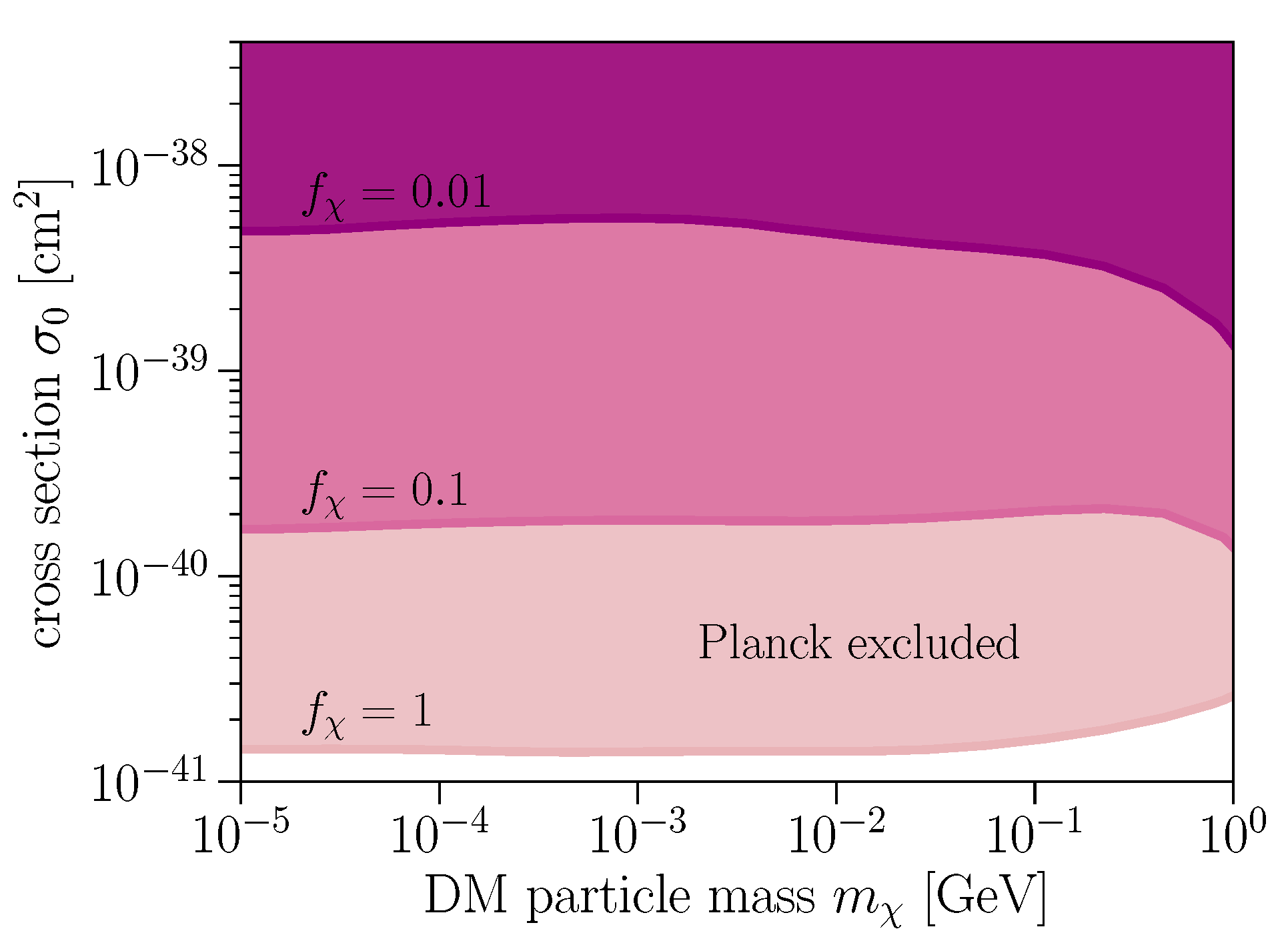
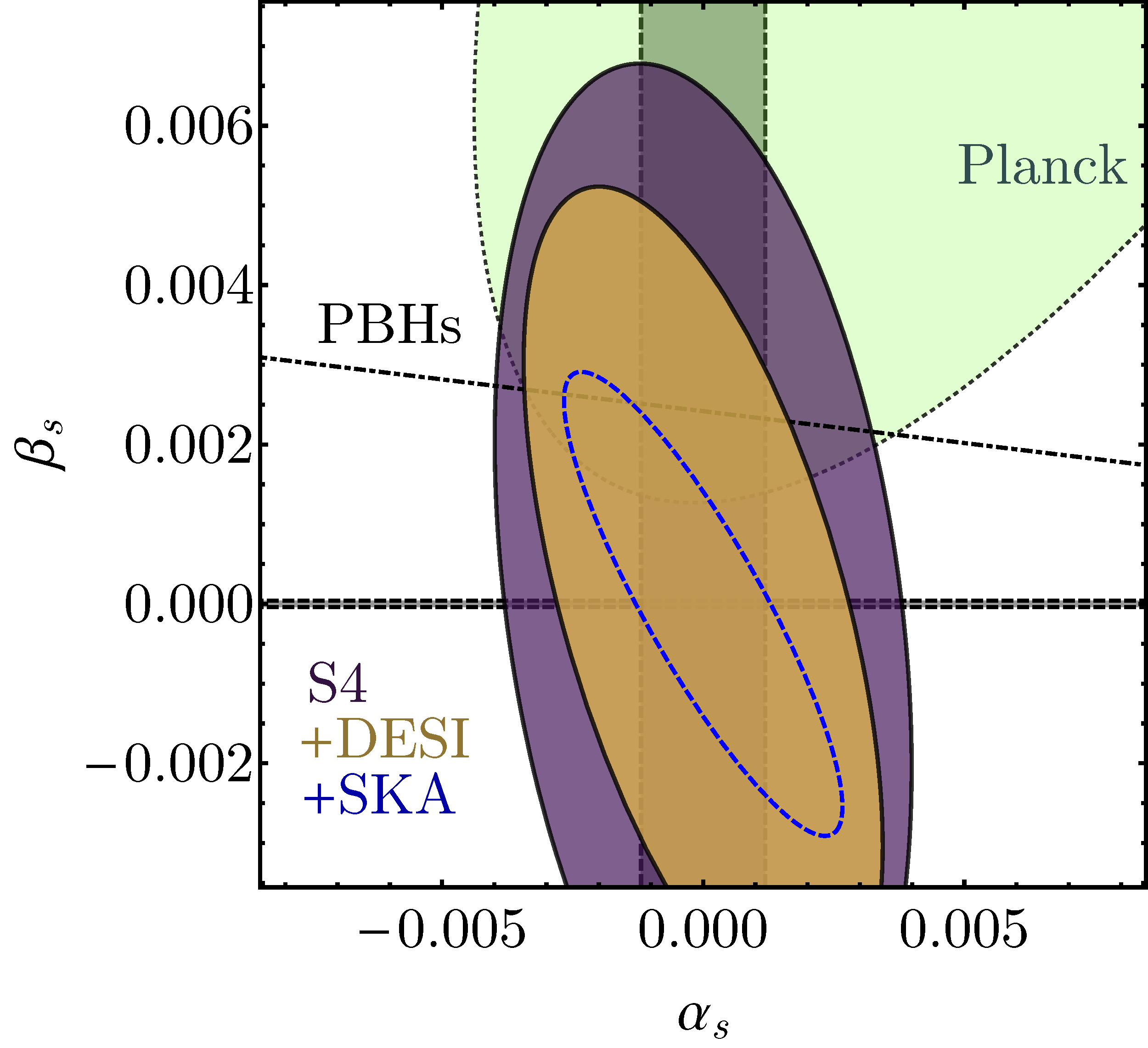
Left: The 95% C.L. excluded region for the coefficient of the DM–proton momentum-transfer cross section as a function of DM mass, obtained by analyzing Planck 2015 data, when the interacting fraction fχ of the total DM energy density is fixed to (from the lightest to the darkest pink): 1, 0.1, and 0.01. From Boddy, Gluscevic, Poulin, Kovetz, Kamionkowski and Barkana (PRD, 2018).
Right: 68% confidence ellipses in the αs − βs plane for the S4 CMB experiment (purple), S4+DESI (yellow), and S4+SKA (blue). We show the current Planck constraint in green. In gray we plot the range predicted by slow-roll single-field inflation. The region above the dash-dotted black line could produce PBHs with masses Mpbh > 1e15 gr, if extrapolated to the smallest scales. From Munoz, Kovetz, Raccanelli, Kamionkowski and Silk (JCAP, 2017).
- Munoz, Grin, Dai, Kamionkowski and Kovetz, PRD (2016): “Search for Compensated Isocurvature Perturbations with Planck Power Spectra”
- Munoz, Kovetz, Raccanelli, Kamionkowski and Silk, JCAP (2017): “Towards a measurement of the spectral runnings”
- Boddy, Gluscevic, Poulin, Kovetz, Kamionkowski and Barkana, PRD (2018): “A Critical Assessment of CMB Limits on Dark Matter–Baryon Scattering: New Treatment of the Relative Bulk Velocity”
- Abadi and Kovetz, PRD (2021): “Can Conformally Coupled Modified Gravity Solve The Hubble Tension?”
Observation
An alternative approach to locating low-contamination sky patches beforehand is to develop means to identify the presence of Galactic dust contamination in CMB maps after they are generated. I introduced a unique such tool which uses tailored estimators to detect statistical anisotropy of the polarization orientation due to the large-scale coherence of the Galactic magnetic field.
Top: A B-mode polarization map, exhibiting the characteristic swirling pattern (with opposite orientation around hot/cold spots). Bottom: A simulated B-mode map with constant polarization orientation, exhibiting a local hexadecapolar anisotropy, i.e. dominated by Fourier modes oriented at 45 degrees w.r.t. the polarization orientation. The departure from statistical isotropy due to a slowly-varying orientation angle can be captured with appropriate statistical estimators and used to identify dust foreground contamination. From Kamionkowski and Kovetz (ARAA, 2016).
- Harvard ITC (invited, 2013): “Cosmic Bandits: Exploration versus Exploitation in Cosmological Surveys”
(talks on this also given at Caltech/OBSCOS, CWRU, Columbia, NYU, Berkeley LBNL, JHU, CITA, IAS/Princeton) - Institut d’Astrophysique, Paris (invited, 2015): “Dealing with Dust Foregrounds in CMB B-mode Observations”
- Kamionkowski and Kovetz, PRL (2014): “Statistical Diagnostics to Identify Galactic Foregrounds in B-mode Map”
- Kovetz and Kamionkowski, New Astronomy (2015): “Cosmic Bandits: Exploration versus Exploitation in CMB B-Mode Experiments”
- Kovetz and Kamionkowski, PRD Rapid Comm. (2015): “Strategy to Minimize Dust Foregrounds in B-mode Searches”
Collaborations
- N. Abazajian et al. (2016): “CMB-S4 Science Book, First Edition”
- F. Finelli et al. (2017): “Exploring Cosmic Origins with CORE: Inflation”
Line Intensity Mapping: Astrophysics and Cosmology at High Redshifts
Why am I interested?
Line-Intensity mapping is an invaluable method to study the universe in between the regimes accessible to galaxy surveys and the CMB. It consists of low-resolution measurements of the fluctuations in the integrated emission of atomic and molecular spectral lines. As reviewed in Kovetz et al. (2017), a Status Report composed following a workshop I organized at JHU, this is an emerging field, expecting a flood of new data from upcoming surveys. I have proposed a wide range of uses of this method, from probing dark matter to measuring star-formation, and continue to explore new ways to exploit its vast potential.
What is it about?
Line-Intensity mapping is an invaluable method to study the universe in between the regimes accessible to galaxy surveys and the CMB. It consists of low-resolution measurements of the fluctuations in the integrated emission of atomic and molecular spectral lines. As reviewed in Kovetz et al. (2017), a Status Report composed following a workshop I organized at JHU, this is an emerging field, expecting a flood of new data from upcoming surveys. I have proposed a wide range of uses of this method, from probing dark matter to measuring star-formation, and continue to explore new ways to exploit its vast potential.
The 21cm HI Transition
Dark Matter-Baryon Scattering: With J. Muñoz, a JHU grad student at the time (now @Harvard), I set out to explore how models in which dark matter interacts with baryons can be probed through their imprint on the 21-cm global temperature and its fluctuations. Naively, one would expect the baryon fluid to cool down as it driven to equilibrium with the colder dark matter fluid. However, we found that a crucial ingredient to include is the relative velocity between the baryons and dark matter, which leads to a heating effect due to the friction between the two fluids, which in some cases is more dominant than the cooling. It also leads to a new large-scale component of fluctuations, on top of the standard 21cm power spectrum. We showed that future experiments can constrain interacting dark matter in mass ranges inaccessible to direct detection experiments, providing excellent motivation for ongoing follow-up works.
Recently, following the EDGES detection of an anomalous 21cm absorption profile at z~17, we investigated in detail the viable parameter space for millicharged dark matter to explain the EDGES signal. We provided a thorough analysis of the effect on the CMB and the 21cm in the strong coupling regime, and derived the most stringent constraints on this model.
Ultra-Light Hidden-Photon Dark Matter: Ultra-light hidden photons provide an appealing candidate for dark matter. These are (light) massive vector bosons that arise naturally in many theoretical setups, and which generically interact with the Standard Model through kinetic mixing with the ordinary photons. Ultra-light hidden-photon dark matter produces an oscillating electric field in the early Universe plasma, which in turn induces an electric current in its ionized component whose dissipation results in heat transfer from the dark matter to the plasma. This will affect the global 21cm signal from the Dark Ages and Cosmic Dawn. In work with Ilias Cholis and David E. Kaplan, we focused on the latter, in light of the reported detection by EDGES of an absorption signal at frequencies corresponding to redshift z∼17. By measuring the 21cm global signal, a limit can be placed on the amount of gas heating, and thus the kinetic mixing strength ε between the hidden and ordinary photons can be constrained. We showed that 21cm at cosmic dawn can place the strongest bounds to date on ε across more then ten orders of magnitude in mass, down to 1e-22 eV.
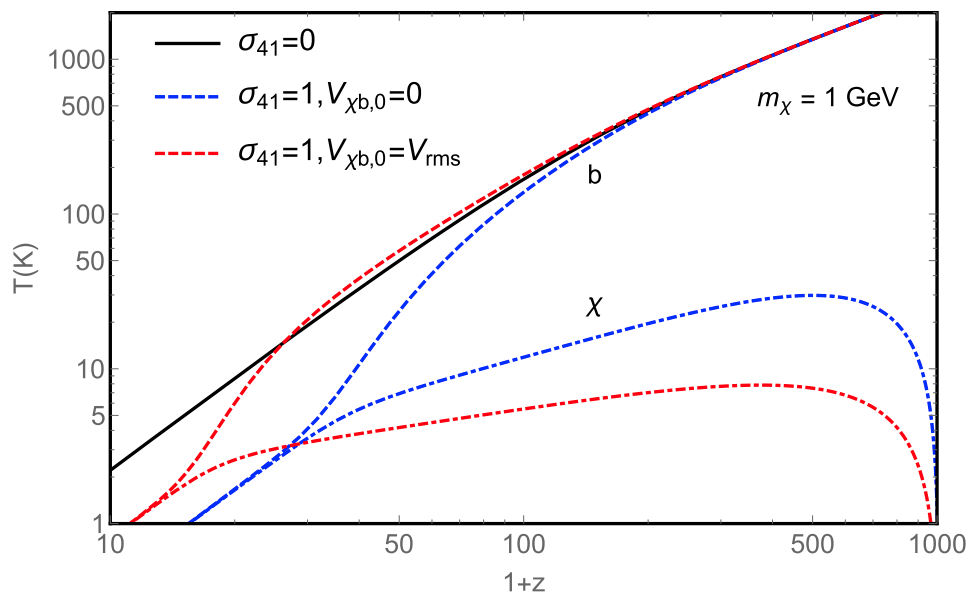
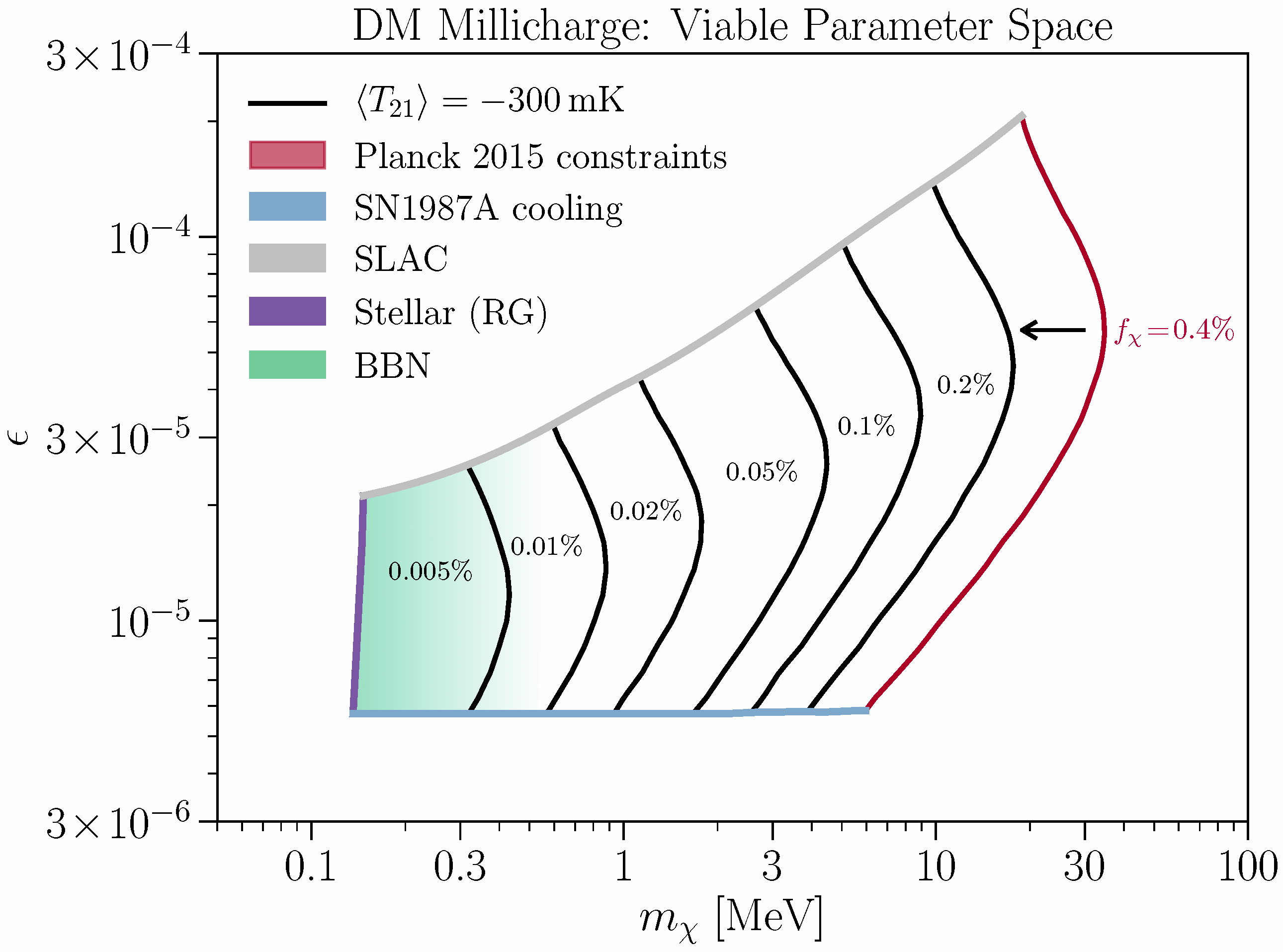
Left: Baryon temperatures (three upper curves) without interactions (solid curve) and when adding interactions (dashed-blue curve for the case where the relative velocity between baryons and dark matter is not taken into account, and red curve when it is), as well as dark-matter temperatures (two lower curves, same scenarios). At late times and for DM mass mχ below a GeV, our prediction was cooling of the baryon gas temperature, which would generate a deeper 21cm absoprtion profile (interestingly matching what EDGES measured a few years later). From Muñoz, Kovetz and Ali-Haïmoud (PRD, 2015).
Right: The viable parameter space for millicharged DM to explain the anomalous EDGES 21cm signal. The allowed region is bound from above by SLAC constraints (gray), from the left by stellar cooling (purple), from below by SN1987A cooling (blue), and from the right by the requirement to cool the baryons enough to yield a 21cm brightness temperature consistent with the EDGES 99% upper bound (black). Contours are shown for several values of the fraction fχ of the total DM that is millicharged; each yields an upper bound on the mass mχ. The rightmost limit is from Planck 2015 (red). A portion ruled out by the Neff limit at BBN, valid below mχ∼me, is sketched (light green). From Kovetz et al. (PRD, 2018).
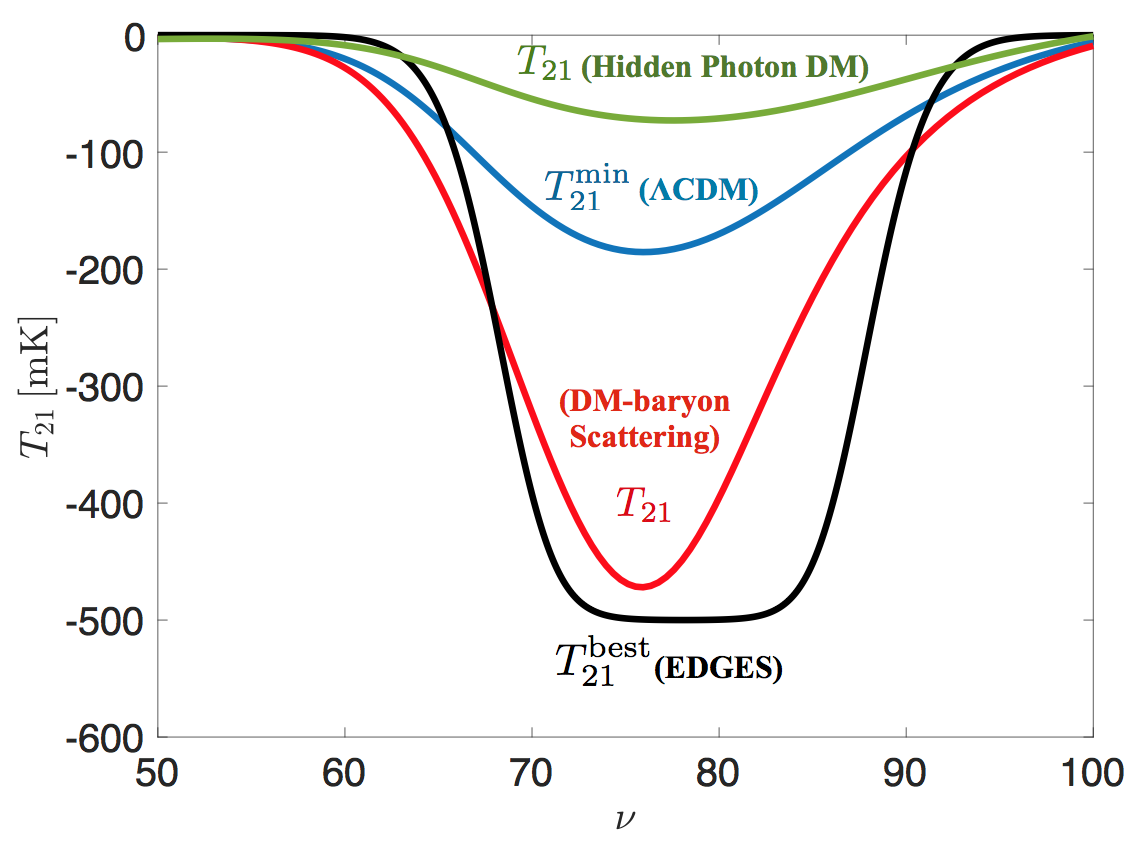
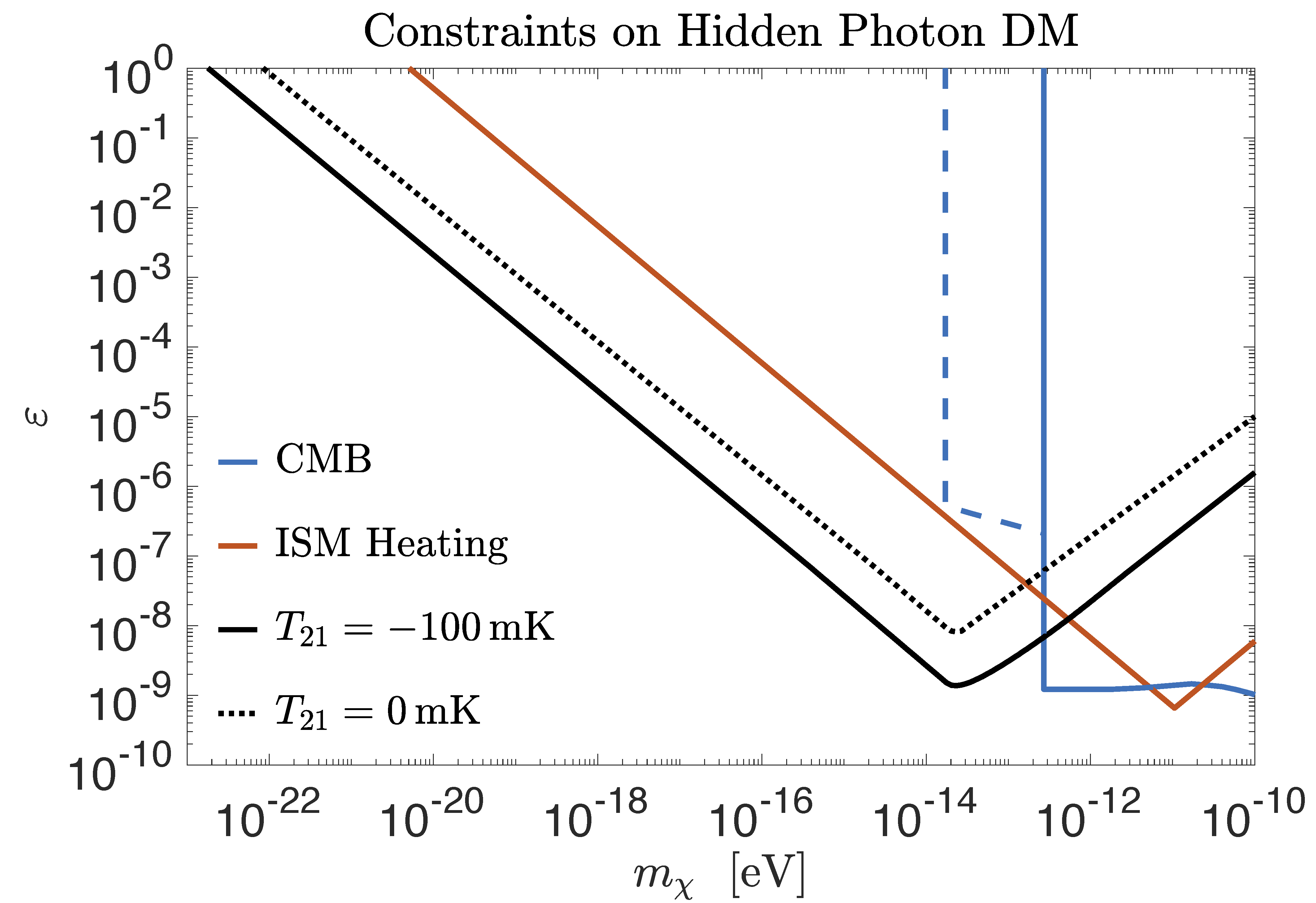
Left: The EDGES Cosmic Dawn 21cm signal (Bowman et al., Nature 2018) is in 3.8σ disagreement with the maximum absorption allowed by ΛCDM. In Kovetz et al. (2018) we present the viable parameter space of the millicharged DM model suggested to explain this anomaly by Barkana (Nature, 2018), based on the calculation in Munoz, Kovetz and Ali-Haımoud (2015). Meanwhile, in Kovetz, Cholis and Kaplan (2018) we show that a robust 21cm detection can place the strongest bounds yet on ultra-light hidden-photon DM (see right panel).
Right: Predominant bounds on the kinetic mixing parameter ε for different hidden-photon DM masses mχ. We show constraints from Milky-Way ISM heating (red) and from the CMB (blue). Our inferred 21cm bounds from requiring that T21 = −100 mK or T21 = −50 mK (black and dashed-black) are two orders of magnitude stronger over ten orders of magnitude in mass and the only ones to penetrate the fuzzy-DM mass range. From Kovetz, Cholis and Kaplan (Submitted to PRL, 2018).
- BCCP Workshop (2013): “Probing localized structures using weak-lensing of 21cm fluctuations”
- Harvard Particle Physics Seminar (invited, 2016): “Cosmological Probes of Dark Matter off the Beaten Path”
- University of Maryland HEP Seminar (invited, 2018): “Dark Matter in Light of the 21cm EDGES Signal”
- “Beyond the Standard Model” Workshop, GGI, Florence, Italy (invited, 2018): “New Cosmological Probes of the Lightest and Heaviest Dark”
- Kovetz and Kamionkowski, PRD 2013: “Galaxy-Cluster Masses via 21st-Century Measurements of Lensing of 21-cm Fluctuations”
- Kovetz and Kamionkowski, PRL 2013: “21-cm Lensing and the Cold Spot in the Cosmic Microwave Background”
- Muñoz, Kovetz and Ali-Haïmoud, PRD 2015: “Heating of baryons due to scattering with dark matter during the dark ages”
- Kovetz, Poulin, Gluscevic, Boddy, Barkana and Kamionkowski, PRD 2018: “Tighter Limits on Dark Matter Explanations of the Anomalous EDGES 21cm Signal”
- Kovetz, Cholis and Kaplan (PRD, 2018): “Bounds on Ultra-Light Hidden-Photon Dark Matter from 21cm at Cosmic Dawn”
- Sarkar, Flitter and Kovetz (PRD, 2021): “Exploring delaying and heating effects on the 21-cm signature of fuzzy dark matter”
Carbon-Monoxide (CO) Intensity Mapping
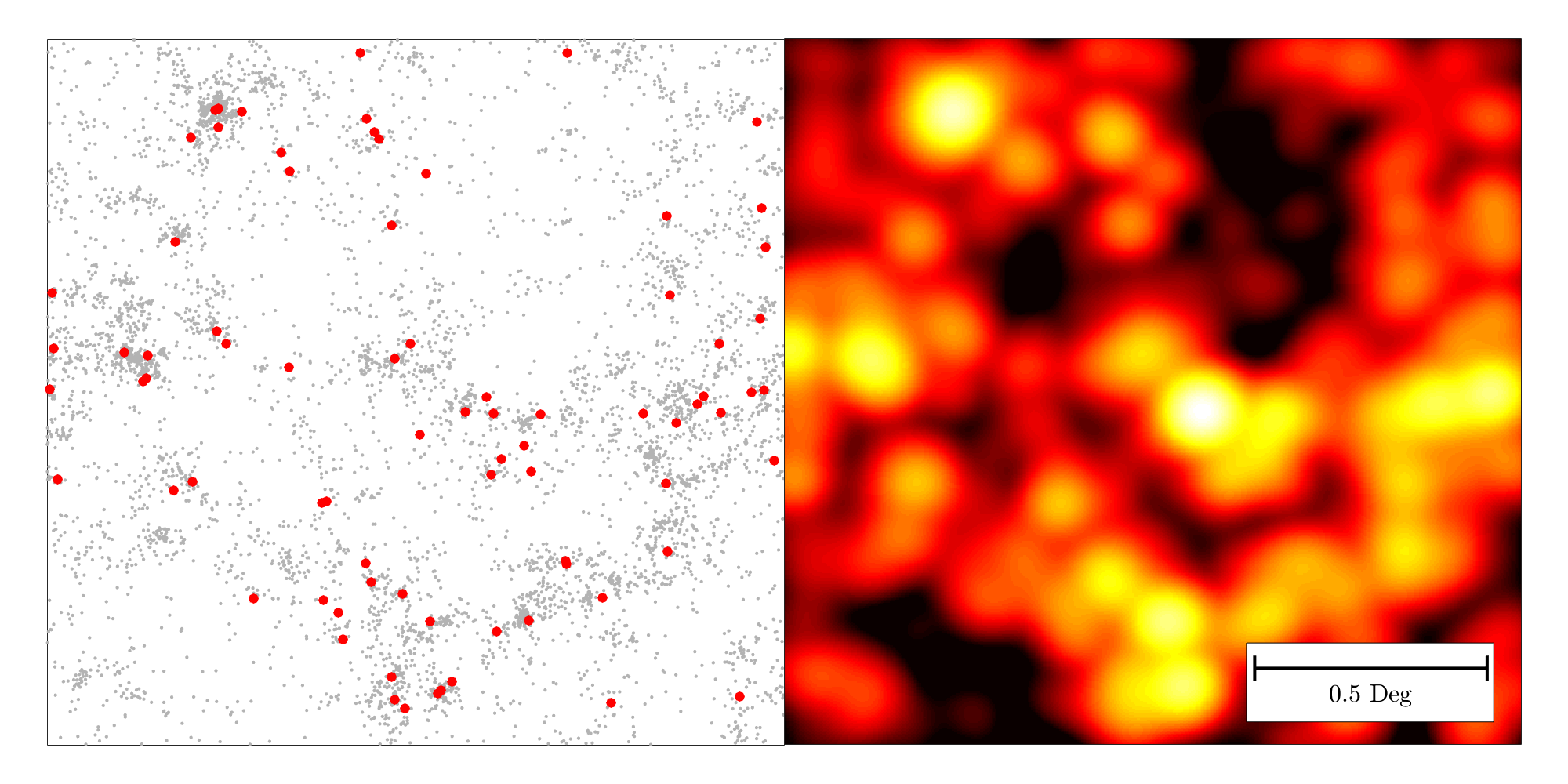
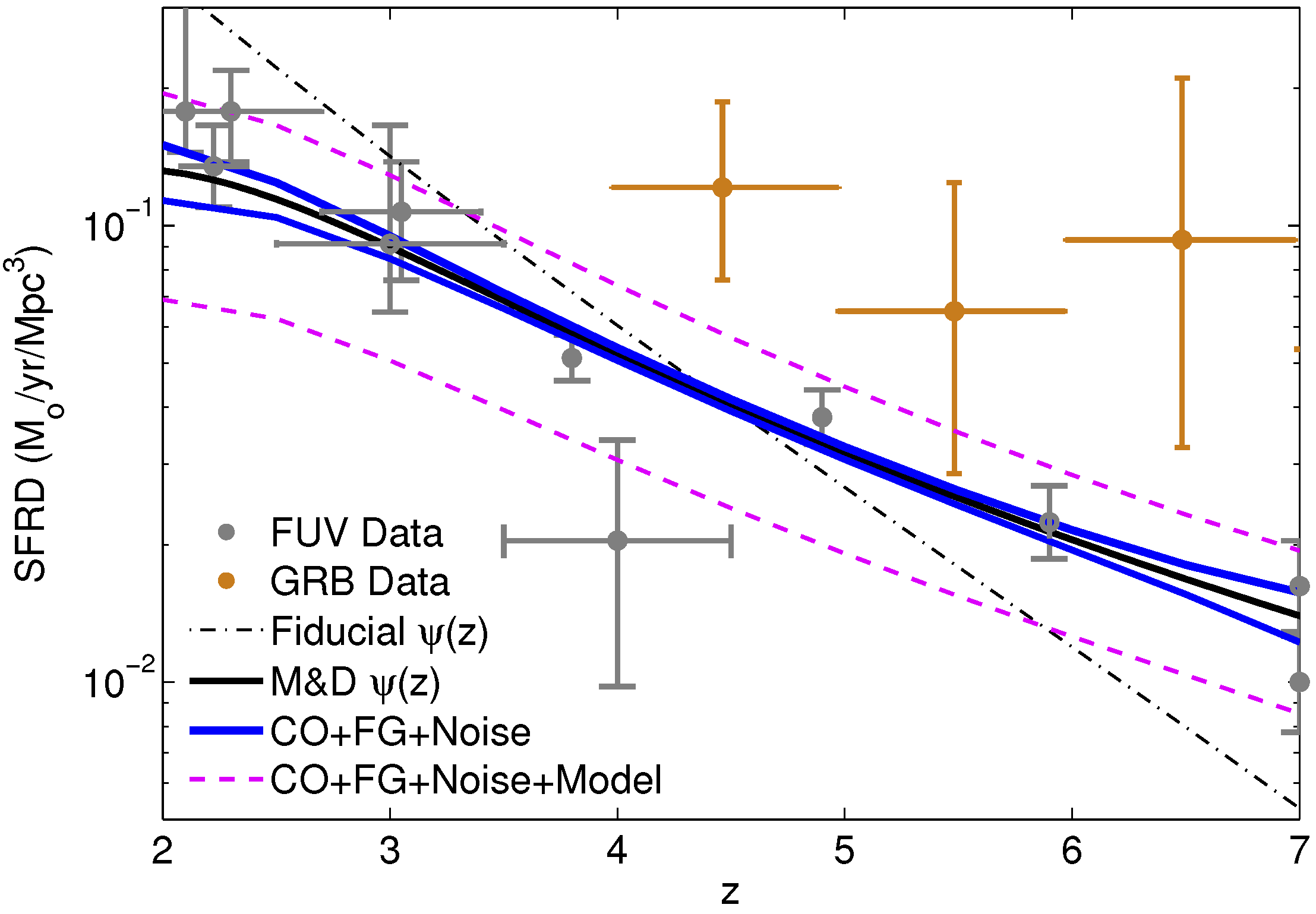
Left: Demonstrating the power of intensity mapping – a simulated 2.5 deg^2 field with galaxy positions (left side) and the corresponding CO intensity map (right side). Luminosities were drawn from a Schechter function model (Breysse, Kovetz and Kamionkowski, 2016). Sources bright enough to detect with 1hr of VLA time are marked in red. In the same 3000 hours of integration over this field required for the VLA detections, a low-cost experiment like COMAP can provide the intensity map on the right. From Kovetz et. al (Physics Reports, in review 2017) .
Right: Comparison between predicted constraints on star formation rate density from CO intensity mapping and from existing FUV (grey points) and GRB (orange points) data. Solid black curve ψ(z) shows fit to FUV data. Blue curves show ±1σ SFRD uncertainty forecast with CO intensity mapping, taking into account foregrounds and noise. Dashed magenta lines encompass a 10% model uncertainty band in the adopted CO-FIR and FIR-SFR relations. From Bryesse, Kovetz and Kamionkowski (MNRASL, 2016) .
- Weizmann Institute (invited, 2016): “Signals and Interlopers: Exhausting Cosmological Information from the Depths of the Observable Universe ”
- Conference, “Cosmological Signals from Cosmic Dawn to the Present”, Aspen, CO (invited review talk, 2018): “Line-Intensity Mapping: (Bird’s-Eye) Theory Review”
- Breysse, Kovetz and Kamionkowski, MNRAS 2014: “Carbon Monoxide Intensity Mapping at Moderate Redshifts”
- Breysse, Kovetz and Kamionkowski, MNRAS 2015: “Masking Line Foregrounds in Intensity Mapping Surveys”
- Breysse, Kovetz and Kamionkowski, MNRAS Letters 2016: “The High Redshift Star-Formation History from Carbon-Monoxide Intensity Maps”
- Breysse, Kovetz, Behroozi, Dai and Kamionkowski, MNRAS 2017: “Insights from Probability Distribution Functions of Intensity Maps”
- Bernal, Breysse, Gil-Marin and Kovetz, PRD 2019: “A User’s Guide to Extracting Cosmological Information from Line-Intensity Maps”
- Bernal, Breysse and Kovetz, PRL 2019: “The Cosmic Expansion History from Line-Intensity Mapping”
- Sato-Polito, Kovetz and Kamionkowski, PRD 2020: “Antisymmetric cross-correlation of line-intensity maps as a probe of reionization”
Collaborations
I then led an effort to develop a document presenting a status report of the line-intensity mapping field following the two workshops. In between, during and after these two workshops, through their presentations, personal writing assignments and feedback on drafts, over 45 scientists (from over 25 institutions) contributed to the 2017 Status Report, while a small writing group was responsible for editing, combining and integrating the individual contributions. The next community workshop will take place in February 2018, at the Aspen Center of Physics.
- Kovetz et al. (Physics Reports, in review 2017): “Line Intensity Mapping: 2017 Status Report”
Gravitational Waves from Mergers of Compact Objects: a Powerful New Probe
Why am I interested?
Gravitational wave signals open up a new window to study the Universe. Black hole mergers in particular are uniquely useful in testing both cosmological models and astrophysical processes. I have thoroughly investigated the relation between gravitational waves and compact dark matter and am working on probing dark energy, as well as stellar-black-hole formation, globular clusters and formation of supermassive black holes.
What is it about?
Gravitational wave signals open up a new window to study the Universe. Black hole mergers in particular are uniquely useful in testing both cosmological models and astrophysical processes. I have thoroughly investigated the relation between gravitational waves and compact dark matter and am working on probing dark energy, as well as stellar-black-hole formation, globular clusters and formation of supermassive black holes.
Primordial Black Hole Dark Matter
In recent celebrated work, my colleagues at JHU and I proposed that the GWs detected by LIGO originated from the coalescence of ~30 M⊙ primordial-BH (PBH) binaries which make up the dark matter. We found the rate of such mergers, dominated by eccentric events in low-mass dark matter halos, to be consistent with LIGO estimates for the merger rate of massive BHs.
How would we detect PBH dark matter?
As illustrated in the figure below (from Kovetz, PRL 2017), we can use an analogy with the detection methods of particle dark matter. Direct detection looks for signatures of direct interactions between standard astrophysical objects and PBHs. Indirect detection examines the signal from “annihilation” of two PBHs, i.e. gravitational waves.
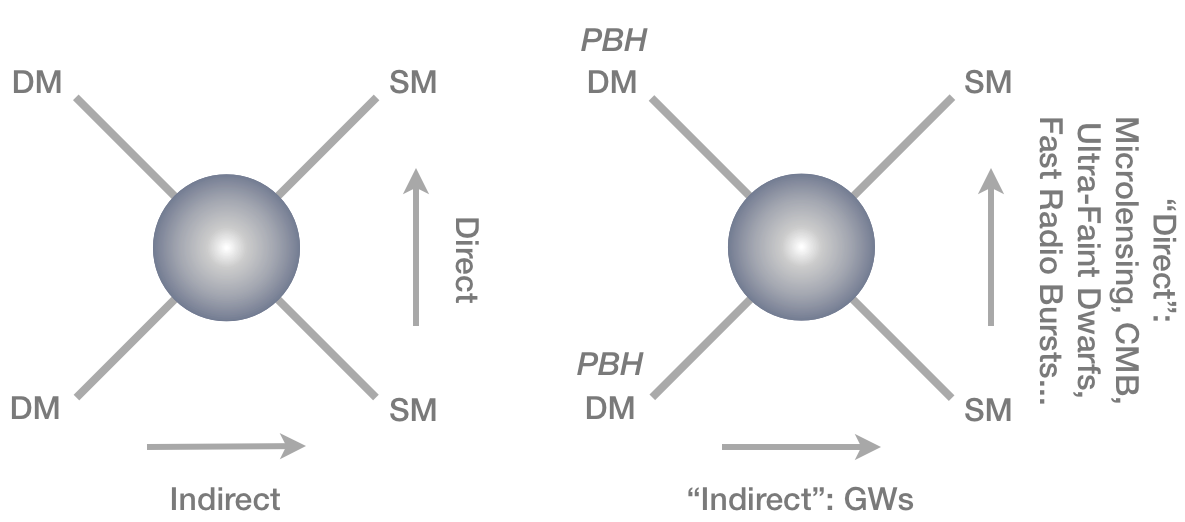
Direct detection: With J. Muñoz, a JHU graduate student at the time (now @Harvard), we proposed a general method to probe compact dark matter by studying Fast Radio Bursts (FRBs) with repeating echoes as a result of strong gravitational lensing by these compact sources, showing that tight bounds can be achieved in the ∼20−100M⊙ mass range with one year of CHIME observations. Future analysis of GRB autocorrelations may lead to even tighter constraints (Ji, Kovetz and Kamionkowski, 2018).
Indirect detection: As in the particle dark-matter case, the challenge is to quantify the standard model background and identify unique features of the desired signal. In Kovetz (PRL, 2017), I examined the indirect detection prospects with LIGO, taking into account the stellar-BH background. With JHU collaborators, we suggested to probe the progenitors of merging binaries based on the characteristically high orbital ellipticity in those formed by two-body capture. Finally, in recent work we considered the merger rate of early-Universe PBH binaries, estimating analytically the effects of interactions with baryons and other dark matter, and showed that LIGO may already place strong constraints on PBHs. Follow up work involves numerical simulations to verify this.
Left: Constraints on the fraction fDM of dark matter in PBHs in the LIGO mass window. Microlensing con- straints shown in cyan; from galactic wide binaries in red; from the lensing magnification PDF of SNe in blue; dynamical constraints from Eri II and ultra-faint dwarfs in green/yellow. Stronger limits are inferred from LIGO O1 results (Ali-Haımoud, Kovetz and Kamionkowski, 2017) and expected soon from null detection of strong lensing of FRBs in CHIME (Munoz, Kovetz et al., PRL 2016).
Right: Future constraints on the fraction of dark matter in PBHs, as a function of mass: Dashed lines correspond to 3σ limits, solid lines are more stringent 5σ limits. Constraints based on the 1D mass distribution shown in red; 2D distribution (tighter, as the noise per bin is smaller) in blue. For 30M⊙, we get fPBH 50% at 5σ (3σ) in the 2D (1D) case. Bands indicate factors 400% (200%) uncertainty in the background (signal) amplitude. From Kovetz (PRL, 2017).
- UPenn Astrophysics Seminar (invited, 2017): “Probing Primordial Black Hole Dark Matter”
(talks on this also given at APS2017/invited-session-talk, UW/Colloquium, UMN, Syracuse, WashU/Colloquium, Fermilab, BNL, USC/Colloquium, PASCOS2018)
- Bird, Cholis, Muñoz, Ali-Haïmoud, Kamionkowski, Kovetz, Raccanelli, Silk, PRL (2016): “Did LIGO detect dark matter?”
- Raccanelli, Kovetz, Bird, Cholis and Muñoz, PRD (2016): “Determining the progenitors of merging black-hole binaries”
- Muñoz, Kovetz, Dai and Kamionkowski, PRL (2016): “Lensing of Fast Radio Bursts as a Probe of Compact Dark Matter”
- Cholis, Kovetz, Ali-Haïmoud, Bird, Kamionkowski, Muñoz and Raccanelli, PRD (2016): “Orbital eccentricities in primordial black hole binaries”
- Kovetz, Cholis, Breysse and Kamionkowski, PRD (2017): “Black hole mass function from gravitational wave measurements”
- Kovetz, PRL (2017): “Probing Primordial Black Hole Dark Matter with Gravitational Waves”
- Nishikawa, Kovetz, Kamionkowski and Silk, submitted to PRD (2017): “Primordial-black-hole mergers in dark-matter spikes”
- Ali-Haïmoud, Kovetz and Kamionkowski, PRD (2017): “The merger rate of primordial-black-hole binaries”
- Ji, Kovetz and Kamionkowski, PRD (2018): “Strong Lensing of Gamma Ray Bursts as a Probe of Compact Dark Matter”
- Sato-Polito, Kovetz and Kamionkowski, PRD (2019): “Constraints on the primordial curvature power spectrum from primordial black holes”
- Unal, Kovetz and Patil (2021): “Multi-messenger probes of inflationary fluctuations and primordial black holes”
- Krochek and Kovetz, PRD (2022): “Constraining Primordial Black Hole Dark Matter with CHIME Fast Radio Bursts”
Astrophysical Black Holes
In Kovetz et al. (2017), I explored how the BH mass function can be probed with LIGO, providing important insights regarding stellar-BH formation (such as the empirically motivated mass gap between Neutron Stars and BHs), progenitor scenarios for merging binaries, wind-driven mass-loss in Wolf-Rayet stars, etc. Based on the simplest assumptions for the stellar-BH mass function—an Initial Mass Function (α=−2.35) power-law and an exponential cutoff—our prediction (with half the data published at the time) for the distribution of detected mergers with BH masses M1,M2 agrees nicely with new data. The prescription we provided for estimating future measurement uncertainty in the mass function and merger rate parameters is being further developed and will enable to place constraints on more detailed modeling of the progenitors, taking into account star-formation history and metallicity evolution, isolated vs. dynamical binary formation, etc.
Left: Our redicted mass distribution of detected stellar-BH mergers. The majority of events will have primary (secondary) masses in the 30−40 M⊙ (20−30 M⊙) range.
Right: LIGO-Virgo events, including mass gap between NS and BHs (Credit: LIGO/Virgo Collaboration).
In recent work with colleagues at JHU, we focused on probing hierarchical mergers in globular clusters (GCs) and the runaway formation of intermediate mass BHs with LIGO, showing how future LIGO measurements can set limits on their occupation fraction in GCs (with implications for their potential to provide supermassive BH (SMBH) seeds). Recent work (Wong, Kovetz et al., 2018) addressed the synergy between ground-based observatories and LISA, showing that information from the former can greatly boost the detection rate of stellar-mass BH mergers in the latter. Essentially, we demonstrated that the ground-based catalog of detected events can be used to filter out spurious noise triggers that show up as the detection threshold of LISA is lowered. We showed that this could yield as much as a factor 4-8 increase in the number of “multi-band” stellar-mass black-hole mergers – i.e., events detected in both a ground-based LIGO-type network and LISA. Multi-band detections (with larger frequency lever arm) will yield better measurements of eccentricity (crucial for distinguishing between binary-formation channels) and enable various tests of modified GR.
Left: Predicted mass distributions of detected stellar-BH mergers (Kovetz et al. 2017) without (blue) and with (red) the inclusion of mergers from runaway formation of intermediate-mass black holes (Kovetz et al. 2018). Advanced LIGO will be able to probe this IMBH formation mechanism.
Right: Boost factor in LISA detection rate of stellar-mass BH mergers using ground-based information (Wong, Kovetz et al. 2018)
- Tel-Aviv University Astro Seminar (invited, 2016): “Gravitational Waves from Merging Black Holes as a Probe of Astrophysics and Cosmology”
- Kovetz, Cholis, Breysse and Kamionkowski, PRD (2017): “The Black Hole Mass Function from Gravitational Wave Measurements”
- Kovetz, Cholis, Kamionkowski and Silk, PRD (2018): “Limits on Runaway Growth of Intermediate Mass Black Holes from Advanced LIGO”
- Wong, Kovetz, Cutler and Berti, PRL (2018): “Expanding the LISA Horizon from the Ground”
- Flitter, Muñoz and Kovetz, MNRAS (2021): “Outliers in the LIGO Black Hole Mass Function from Coagulation in Dense Clusters”
Happy News!
April 2022
Ely Kovetz is awarded the 2022 Krill Prize by the Wolf Foundation
February 2022
Sarah Libanore wins an Azrieli Postdoc Fellowship, to start in January 2023
January 2022
Jordan Flitter wins the Kreitmann School Negev Ongoing PhD Fellowship
June 2021
Ely Kovetz is awarded the 2021 Toronto Prize for Excellence in Research
April 2021
Caner Unal wins the Israel Academy of Sciences CHE Postdoc Fellowship