Research Interests
Current Interests
Long-range, Non-local Switching of Spin Textures in a Frustrated Antiferromagnet
Antiferromagnetic spintronics is an emerging area of quantum technologies that leverage the coupling between spin and orbital degress of freedom in exotic materials. Spin-orbit interactions allow spin or angular momentum to be injected via electrical stimuli to manipulate the spin texture of a material, enabling the storage of information and energy. In general, the physical process is intrinsically local: spin is carried by an electrical current, imparted into the magnetic system, and the spin texture then rotates. The collective excitations of complex spin textures have rarely been utilized in this context, even though they can in principle transport spin over much longer distances, using much lower power. In this study, we show that spin information can be transported and stored non-locally in the material FeχNbS2. We propose that collective modes leverage the strong magnetolastic coupling in the system to achieve this, revealing a novel way to store spin information in complex magnetic systems.
Metastable Slidtronics Switching in Bulk 1T-TaS2
The control of correlated materials by direct electronic stimulus enables coupling to nontrivial degrees of freedom. Specifically, the ultra-fast insulator-metal transition activated in 1T-TaS2 by various stimuli methods serves as a perfect platform to study the response of charge order to injection of confined oriented currents. In this study, we show in bulk 1T-TaS2 that the coupling of DC electrical pulses to various directions of the charge density wave results in nonvolatile metastable resistance states. By fabricating multiple pulse channels, we were able to effectively produce a resistance switch susceptible to the activated current direction. A crystallographic directional response in the form of charge density wave sliding motion throughout the insulating to metal transition is proposed and demonstrated theoretically. The ability to systematically control the charge order stacking configuration opens a path for novel studies in correlated layered materials together with new opportunities for modern technological devices.
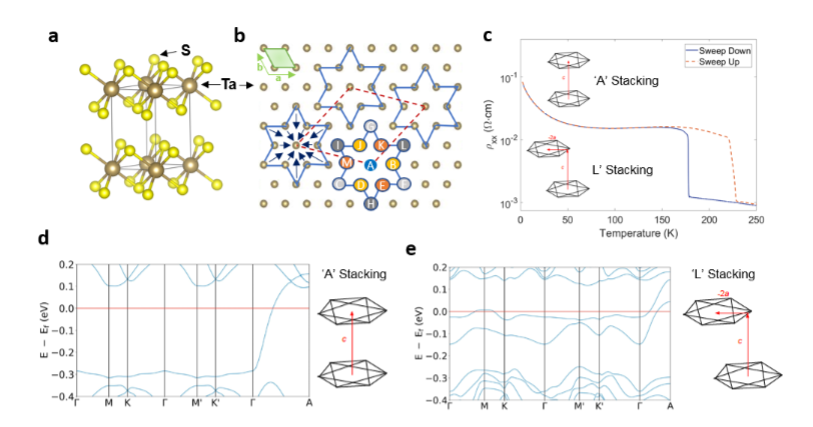
Article in progress.
Recent Grants Awarded
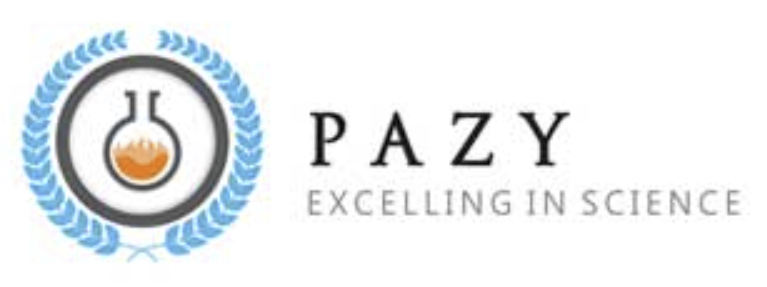
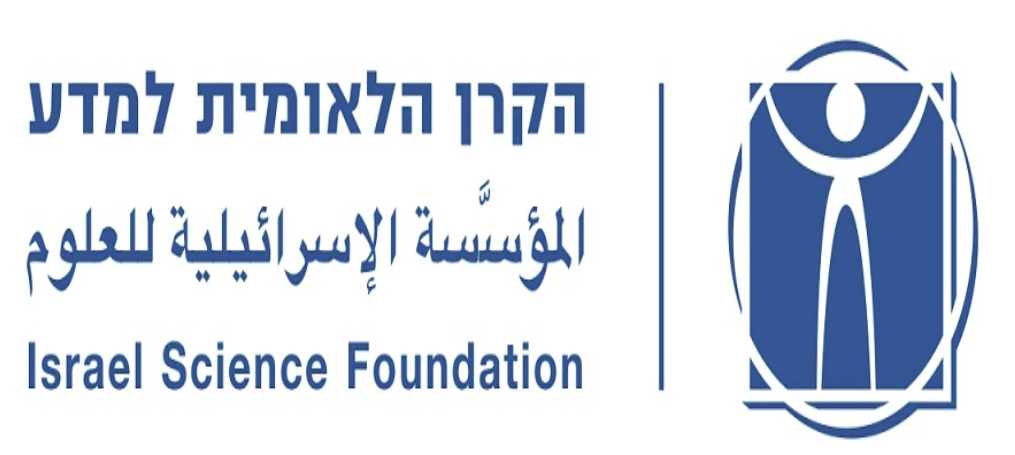
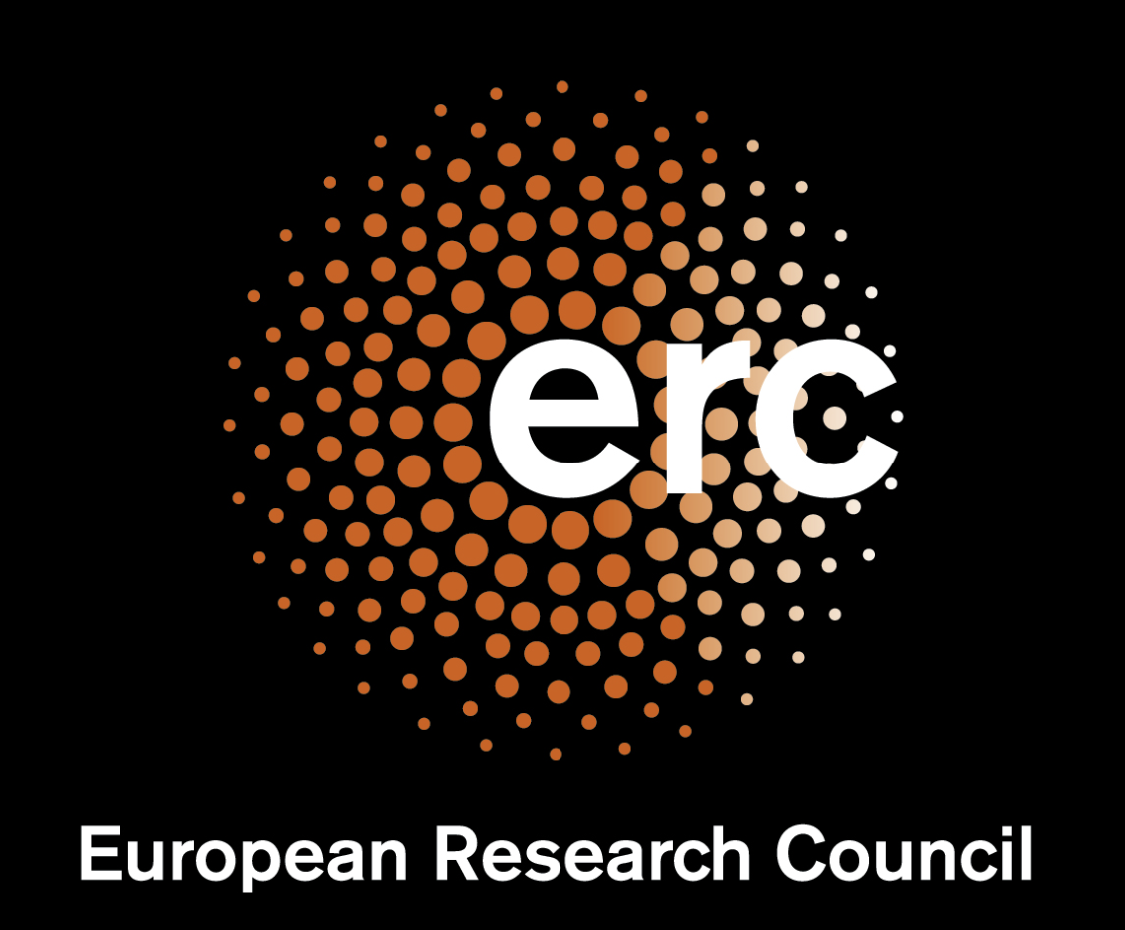
In the News
Disordered exchange is biased
The magnetic properties of intercalated metal dichalcogenides are dramatically afected by small crystal imperfections, potentially providing design principles and materials for spintronic devices.
by Minhyea Lee, in Nature Physics
Researchers demonstrate the potential of a new quantum material for creating two spintronic technologies.
by Ingrid Fadelli
Exchange bias set in a spin-glass phase could arise in a disordered antiferromagnet.
by Bob Yirka
Past Studies
Strong correlations elucidate the electronic structure and phase diagram of LaAIO3/SrTiO3 interface
The interface between the two band insulators SrTiO3 and LaAIO3 has the unexpected properties of a two-dimensional electron gas. It is even superconducting with a transition temperature, Tc, that can be tuned using gate bias Vg, which controls the number of electrons added or removed from the interface. The gate bias-temperature (Vg, T) phase diagram is characterized by a dome-shaped region where superconductivity occurs, that is, Tc has a non-monotonic dependence on Vg, similar to many unconventional superconductors. Here, we report, the frequency of the quantum resistance-oscillations versus inverse magnetic field for various Vg. This frequency follows the same non-monotonic behaviour as Tc; a similar trend is seen in the low field limit of the Hall coeficient. We theoretically show that electronic correlations result in a non-monotonic population of the mobile band, chich can account for the experimental behaviour of the normal transport properties and the superconducting dome.
Electrical switching in a magnetically intercalated transition metal dichalcogenide
Advances in controlling the correlated behaviour of transition metal dichalcogenides have opened a new frontier of many-body physics in two dimensions. A field where these materials have yet to make a deep impacts is antiferromagnetic spintronics – a relatively new reseacrh direction promising technologies with fast switching times, insensitivity to magnetic perturbations and reduced cross-talk. Here, we present measurements on the intercalated transition metal diacholcogenide Fe½NbS2 that exhibitis antiferromagnetic ordering below 42K (refs.). We find that remarkably low current densities of the order, which can be detected through changes in the sample resistance, demonstrating its use as an electronically accessible antiferromagnetic switch. Fe½NbS2 is part of a larger family of magnetically interca;ated transition metal dichalcogenides, some of which may exhibit switching at room temperature, forming a platform from which to build tuneable antiferromagnetic spintronic devices.
Exchange bias due to coupling between coexisting antiferromagnetic and spin-glass orders
Exchange bias is a property of widespread technological utility, but its undelying mechanism remains elusive, in part because it is rooted in the interaction of coexisting order paramaters in the presence of complex magnetic disorder. here we show that a giant exchange bias housed within a spin-glass phase arises in a disordered antiferromagnet. the magnitude and robustness of the exchange bias emerges from a convolution of two energetic landscapes, namely the highly degenerate landscape of the spin glass biased by the sublattice spin configuration of the antiferromagnet. The former provides a source of uncompensated moment, whereas the latter provides a mechanism for its pinning, which leads to the exchange bias. Tuning the relative strengths of the spin-glass and antiferromagnetic order parameters reveals a principle for tailoring the exchange bias, with potential applications to spintronic technologies.
Evolution of the Fermi Surface of a doped topological insulator with carrier concentration
In an ideal bulk topological insulator (TI) conducting surface states protected by time-reversal symmetry enfold an insulating crystal. However, the archetypal TI, Bi2Se3, is acyually never insulating; it is in fact a relatively good metal. Nevertheless, it is the most studied system among all the TIs, mainly due to its simple band structure and large spin-orbit gap. Recently, it was shown that copper intercalated Bi2Se3 becomes superconducting and it was suggested as a realization of a topological superconductor. Here we use a combination of techniques that are sensitive to the shape of the Fermi surface (FS): the Shubnikov-de Haas effect and angle-resolved photoemission spectroscopy to study the evolution of the FS shape with carrier concentration, n. We find that as n increases, the FS becomes two-dimensional-like. These results are of crucial importance for understanding the superconducting properties of CuxBi2Se3.